In an age of corporate social sustainability and rising emphasis on preventing climate change, it’s no surprise that concern about pollution extends to the stars. The initial launches of the Starlink and OneWeb mega-constellations have reignited debate on how orbital debris mitigation and removal should be addressed. This article offers insight into the costs of cleaning up the space environment, providing a valuable baseline for policy makers and industry stakeholders.
Introduction
Ever since the first satellite was launched in 1957, humanity has been slowly cluttering space with debris. Discarded rocket cores, defunct spacecraft, even the occasional Tesla Roadster, now fill the space environment and are creating challenges for the world’s future spaceflight ambitions. While debris is heavily concentrated in only a handful of popular orbits, the sheer magnitude of the problem is enough to frighten anyone planning to operate in near-Earth-space[1].
An estimated 20,000+ pieces of debris larger than the size of a softball are currently being tracked in orbit. Even more are too small to be seen by modern equipment – some estimates place the total number of orbital debris in the tens of millions[1]. The vast majority of these debris are uncontrollable; even if they can be seen, there is little that can be done to move them out of the way. Given that even tiny pieces of debris can be catastrophic, the compounding build-up of space junk is worrisome, to say the least.
Putting Orbital Debris into Context
Lawmakers, space advocates, and others in the industry are understandably concerned over the growing amount of debris in orbit, but is the risk of collision that substantial? The reality is space is big – there is ample room for debris to float around for decades and never encounter another object.
Consider, for example, if we were to take all of the debris in a given orbit and equally space it out across the Earth starting in New York City. In this scenario, the next closest object in LEO, on average, would be almost 100 km away in Philadelphia. In MEO, that same object would be in Washington DC. By the time we reach GEO, it would be in the United Kingdom!
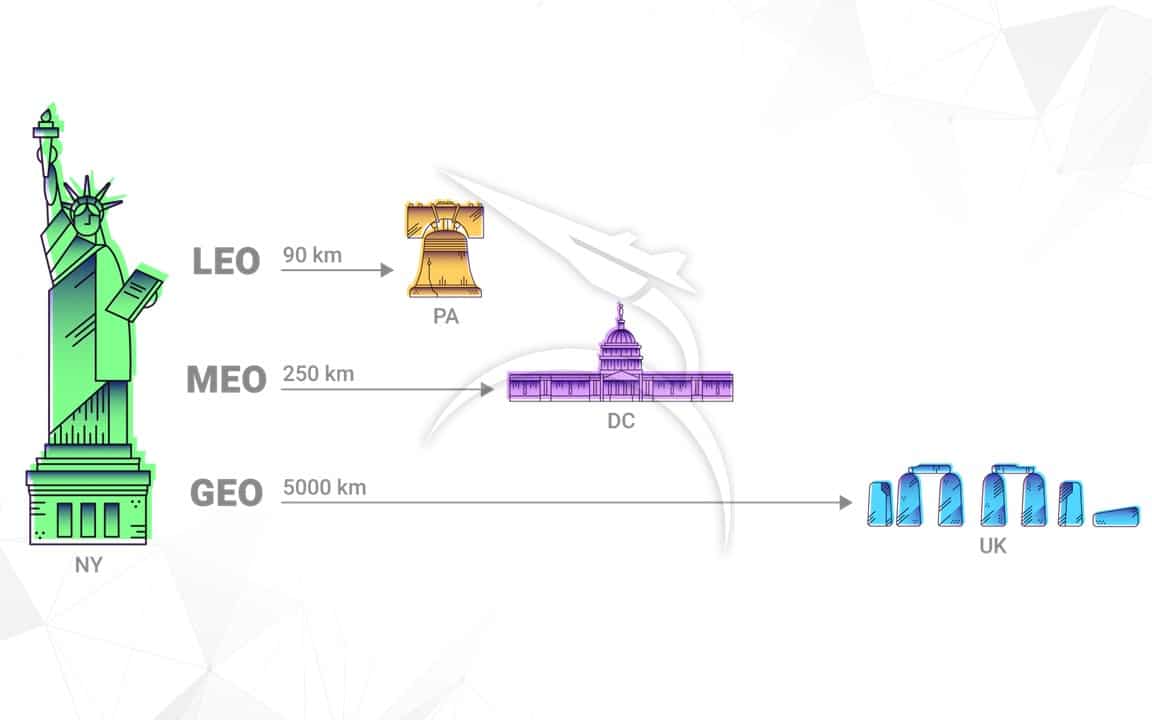
Realistically, the calculation it isn’t quite that simple as objects are not uniformly distributed. Impact events create highly clustered debris clouds, and individual objects are not necessarily traveling in the same direction. Not to mention, distance is relative. It’s a lot quicker to go from New York to Philadelphia when objects are moving 20,000+ mph in space, than by a train on the ground. Still, the chances of an in-orbit collision are about 1 in 10,000 during a satellite’s lifetime[2]. By comparison, from a terrestrial perspective, you are more likely to be involved in an auto accident (about 1 in 40) in a single year, than a satellite is to collide with another object its entire lifetime[3]. So why is it, that orbital debris attracts so much attention by policy makers, commercial operators, and scientists alike?
The Cascade Effect
The issue lies in the compounding impact of space debris. While the risk of an individual collision is low, each collision exponentially increases the likelihood of another collision – a phenomenon known as the Cascade Effect or Kessler Syndrome[4]. In just 60 years, humanity has gone from a single piece of debris, to millions of objects floating in near-Earth-orbit. As more debris begins to occupy the orbital environment, there are more chances for collisions, which in turn will further increase the amount of debris. This scenario creates a vicious cycle that is difficult to stop. Exhibit 2 details the frightening potential of the Kessler Syndrome if it is allowed to progress unchecked.
Exhibit 2. The Kessler Syndrome (Video)
While any piece of orbital debris has the potential to be lethal, not all space junk is created equal. Different types of objects pose different threats. For example, smaller objects are often the cause of a collision, but the larger object they crash into are the source of the majority of debris[5].
Type | Characteristics | Hazard |
Shrapnel | Untracked, over ~1 cm, 98% of lethal objects | Primary threat to satellites; too small to track and avoid, too heavy to shield against |
Hubcaps | Tracked, >10 cm, <2 kg, 2% of lethal objects | Primary cause of most avoidance maneuvers for operational satellites |
Cars | Tracked, over 2kg, <1% of lethal objects | Primary source of new shrapnel; 99% of the collision area and mass |
As seen in the above table, ‘Cars’ present the largest collision area – nearly four times the size of the next category (‘Hubcaps’). Cars make up over 90% of the target area in orbit, despite being less than 1% of the total objects[5]. Because of their size and target area, Cars running into Cars is actually the biggest driver of new debris. The 2009 Iridium-33/Kosmos-2251 incident highlights the particularly dangerous nature of these types of collisions. Even with the satellites’ relatively small size (950 kg and 560 kg, respectively), this impact created an estimated 2,000 new pieces of space debris[6]. Avoiding these incidents in the future is critical to both reducing the number of space debris and, more importantly, preventing the Cascade Effect from ever becoming a reality. The best way to do this is ensuring satellites are effectively deorbited at their end-of-life, preferably as quickly as possible.
A New Era for Orbital Debris Policy
Legislators are not dull to the issues posed by the growing amount of ‘space junk’ in orbit, nor have they been silent on the topic. As early as the 1980s, National Space Policy directives were instructing government agencies and the private sector to minimize the creation of space debris[7]. In fact, nearly every president since has made at least some mention of orbital debris in their Presidential Policy Directives. Exhibit 3 depicts a timeline of the major orbital debris-related policy announcements in the Untied States.
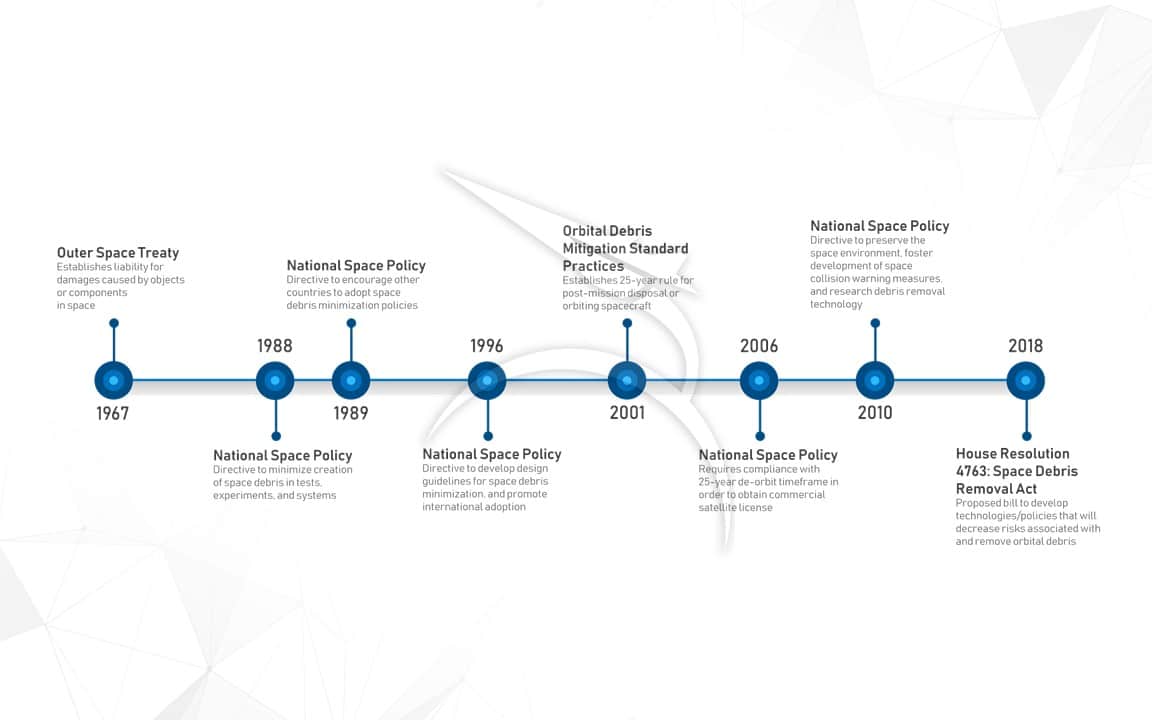
While these space-focused directives have provided guiding objectives and deadlines over the years, they have historically been largely non-binding. A lone exception is the enactment of post-mission disposal rules in the late 2000s, which effectively installed a 25-year cap on orbital lifetime for new satellites. Moving forward, the onset of megaconstellations and the potential for as many as 20,000 new satellites to be launched in the next 10 years are causing policy makers to finally begin considering substantive, enforceable orbital debris legislation[8].
In the past, legislative focus has been concentrated almost exclusively on orbital debris mitigation strategies. Understandable, given that to-date organizations have had little recourse for any type of active debris removal. Over the past few years, however, advancements in proximity operations and autonomous robotics have presented a new path forward for the industry. Companies like Altius Space Machines, Maxar, Northrop Grumman and others have begun working on a critical enabling technology: satellite servicing[9].
The primary market for satellite servicing lies in upgrading, repairing, and extending the life of satellites, but a smaller subset of the market is interested in actively deorbiting spacecraft[10]. Some companies, like Astroscale, are focused exclusively on leveraging this new technology for the purposes of debris removal. As these new capabilities come online, the legislative approach has shifted towards a multi-faceted strategy that includes both mitigation and removal strategies[8].
In early 2018 the Committee of Science, Space, and Technology was introduced to H.R. 4763, which advocated for technology and policy that would “decrease the risks associated with and remove orbital debris”. Although the bill didn’t establish specific statutes, it is one of the first instances of policy makers targeting both debris mitigation and removal[8]. As the conversation around appropriate policy levers continues to evolve, it is critical to arm legislators with the information they need to establish productive policies for reducing the amount of space debris.
Establishing Effective Orbital Debris Removal Policies
SpaceWorks research finds that most satellite operators are responsible corporate citizens, but there are still some firms that have shown a propensity to skirt regulations when they may not be in the company’s financial interest[11]. As orbital debris regulations begin to evolve into more substantive, binding policies, it will be critical to ensure that the temptation for such bad behavior is limited. Legislative commitment that puts in place concrete penalties for those actors that don’t adhere to the rules and/or creates incentives for those that do, is an important component to effectively reducing the amount of space debris.
Motivating industry to act in the best interest of a public good is a delicate balance. Set penalties for externalities too high and you may stifle innovation. Set them too low and you might find that organizations ignore them all together. To aid policy makers in understanding appropriate incentive levels for encouraging self-removal of orbital debris, SpaceWorks investigated the costs associated with such activities.
SpaceWorks considered a scenario in which a small spacecraft servicer performed dedicated deorbit missions for satellites in LEO. Mission operations, fuel, hardware, and business support costs were all modeled to understand the full picture of what it would take to complete such a mission. Exhibit 4 depicts the average estimated cost for actively deorbiting satellites at different altitudes. At these price points, organizations would be indifferent to paying the regulatory fine or contracting a 3rd party to deorbit their satellite. Penalties any lower than this figure are likely to result in organizations simply taking the fine, as it would be more cost effective than bringing down the spacecraft.
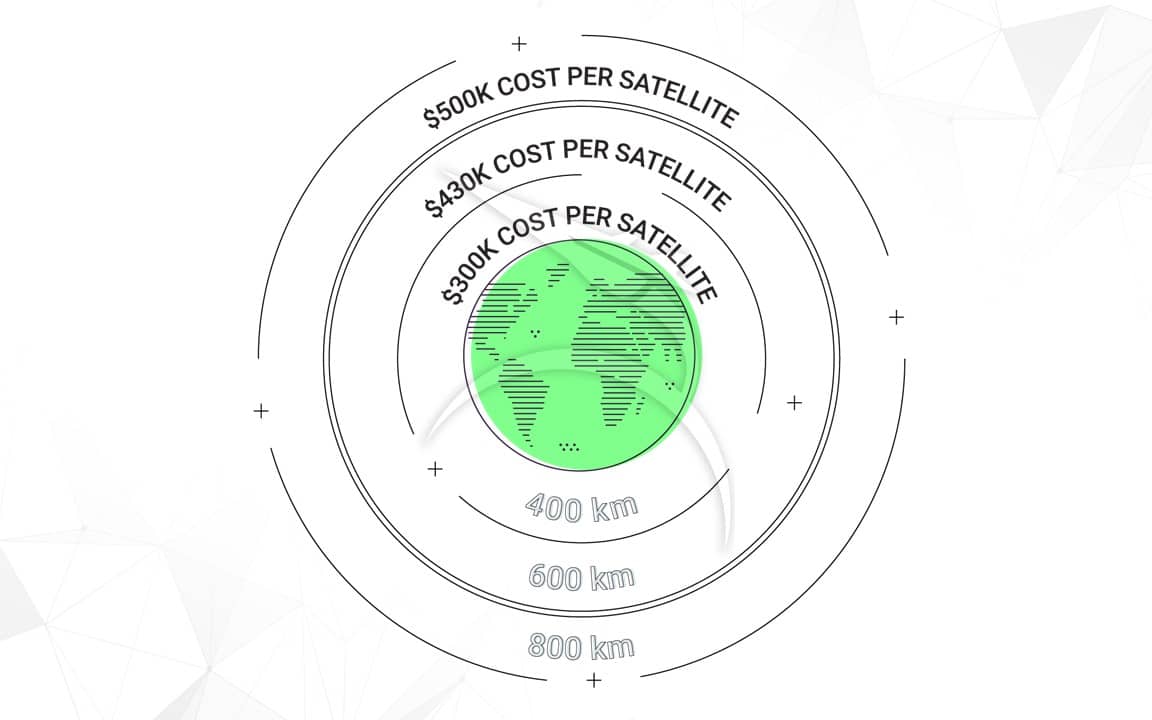
A key finding of this research was that overall deorbit costs are heavily driven by mission duration. Operations and amortized hardware costs were directly linked to the length of time required to complete a sortie. As duration itself is primarily driven by satellite weight and altitude, it can be useful to look at total cost as a function of these two factors. Exhibit 5 depicts estimated deorbit costs by satellite size and altitude.
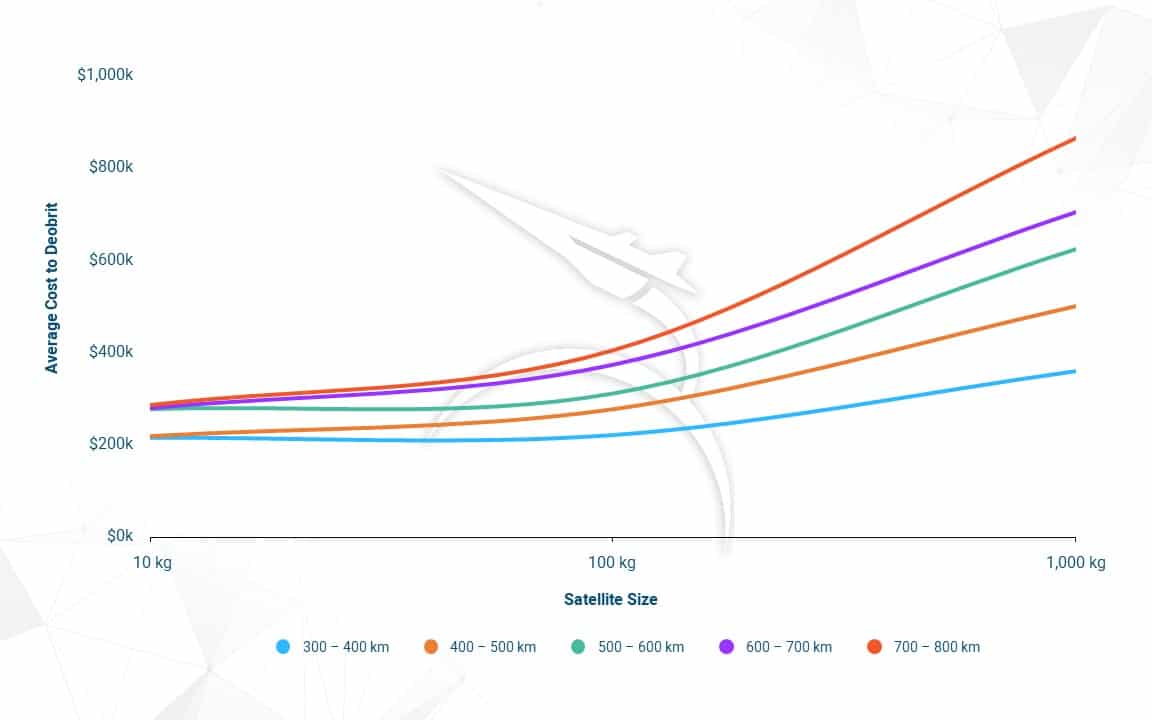
As seen in Exhibit 5, there is a significant change in cost depending on both the satellite’s size and altitude. For a 1000 kg medium-class satellite, the mission cost is as much as 200% more expensive than a CubeSat-class satellite. Following a similar trend, at an 800 km Sun-synchronous orbit, a satellite is up to 140% more expensive to deorbit than an equivalent sized satellite in a 400 km ISS orbit.
It is interesting to note that there is break-point at the 500 km altitude where average costs increase by as much as 25%, regardless of the satellite size. The cause of this step-function increase is due to higher altitudes being associated with additional hardware costs. Above the 500 km range, increased performance requirements dictated additional non-recurring and spacecraft production expenditures which, in-turn, created higher mission costs.
Combining both altitude and size, we find that the difference between deorbiting a CubeSat-class satellite in a 400 km ISS Orbit and a 1000 kg medium-class satellite could be as much 300%. These findings indicate that a tiered incentive approach based on the satellite’s physical and orbital characteristics, rather than a one-size-fits-all strategy, may be necessary. Intuitively, this seems to make sense – the larger the satellite, the more potential debris that might be generated during a collision, hence, the larger the penalty or incentive. Additionally, varying incentive schemes by altitude may be an effective way to de-clutter the more crowded orbits.
It is worth noting, deorbit pricing is highly sensitive to the deorbiter/servicer’s overall utilization. This analysis assumes a 100% utilization rate, which may or may not be a realistic depiction of the number of deorbit missions the market requires. The lower the utilization rate, the higher individual mission costs will be, as they will share a larger portion of amortized costs. A hybrid approach that combines deorbit missions with other types of satellite servicing may necessary to fully occupy the servicer’s time in orbit and create a sustainable business model.
Conclusions
The growing congestion of near-earth orbit offers significant challenges for humanity’s long-term space ambitions, but the emergence of satellite servicing technologies is spurring a new era of possibilities for debris clean-up. As legislators begin to take a more active role in the space environment, it is critical that they push towards a regulatory future that emphasizes debris removal, as well as mitigation. Focus should be on eliminating large objects in space, rather than smaller ones, as these are the greatest contributors to catastrophic scenarios, such as the Cascade Effect.
It appears that using a small spacecraft servicer to actively deorbit satellites is not all that cost prohibitive. While at the low-end of the market (i.e., CubeSat-class), the cost is roughly equivalent to the satellite itself, at the higher-end (i.e., medium-class) it is only a small fraction of total satellite cost (less than 10%). Alternative approaches, such as native deorbit systems or detachable devices could potentially lower the costs for satellite disposal even further, making debris removal activities even more accessible.
From penalties to cap-and-trade systems to bounty programs, there are a variety of legislative programs that can be implemented to incentivize industry actors. The ultimate goal of such policies should be to encourage users to rapidly dispose of their satellites once they reach end of life. Establishing a baseline for deorbit costs provides a useful frame-of-reference for policy makers as they begin to consider new orbital debris legislation.
Certainly, the costs of active debris removal activities will vary based on the technology and approach being used, but the order of magnitude is likely to remain the same as the figures shown here. Cost estimates such as these should serve as guides for policy makers as they begin developing incentive structures to preserve the shared public good that is outer space.